Spacecraft production and operation are very expensive with typical program costs amounting to
hundreds of millions of pounds. Mission costs may be divided into three main phases, each
contributing a significant proportion of the total: the design and construction of the spacecraft,
the launcher and launch program costs, and the cost of operations over the spacecraft life.
The main driving factor is the cost of spacecraft launch. Launchers are extremely expensive to
build and use. A guided rocket, capable of delivering a spacecraft into orbit is an extremely large,
complex, multi-stage device, subject to an extreme range of temperatures and burning highly
corrosive and hazardous fuels. Guidance is complex, the launcher providing huge amounts of thrust in
one axis, while also controlling the other axes to direct the rocket into the correct orbit. Launch
opportunities are often limited by season or by the need to clear the vicinity of the rocket launch
site and flight path. Launchers must be designed to be as highly reliable as possible. In the event
of failure, even if another spare spacecraft exists, another launch opportunity may not exist for
months or years. Despite this, launch failures are surprisingly common: 8% of all spacecraft fail to
start operations (and 11% of those destined for high orbit). Current launch insurance policies cost
about 13B17% of the spacecraft replacement value and
relaunch. While competition for business between launch-vehicle consortia is fierce, with European,
Japanese, Russian, Chinese and American launchers all competing for business, no one has yet been
able to improve significantly launch reliability, reduce launcher cost and offer a rapid service.
Russian and Chinese systems are less expensive than their western counterparts (Chinese rockets
quoted as 30% cheaper), but their reliability and orbital injection precision has been questioned.
Arianespace’s Ariane 4 launcher now receives favourable insurance treatment because of its
90.1% success rate, but has only managed a maximum of 8 launches in one year. The same companies
recent launch failure on the maiden flight of the new Ariane 5 rocket, only illustrates the
difficulties of making vehicles reliable.
The cost of the launch has implications for the design of the spacecraft. With the exception of
communications missions requiring constellations of spacecraft, missions (and in particular, science
missions) tend to be unique—a single spacecraft being launched to carry out a particular
experiment or task, with no spare or alternative mission to carry out the same task in case of
failure. If a spacecraft fails then the mission is unlikely to be repeated for years, if ever.
Spacecraft must therefore be highly reliable, first surviving the harsh vibration environment of
launch, and then operating successfully for long lifetimes without servicing and despite large
radiation doses. The design and manufacturing processes must be rigorous, ensuring the correct
operation of the spacecraft the first and only time it will be used. The systems and electronics are
analysed down to a component level to ensure that the failure of any particular constituent part
will not jeopardise the longevity of the whole mission. Design concepts must also be continually
re-examined to verify their validity. Construction is to the highest quality with no expense spared.
The current design trend is towards simple, well-tested, conservative design, producing large,
heavy systems that ignore new technologies. This further spirals mission costs—the size and cost
of the launcher being proportional to the size and mass of the spacecraft.
High spacecraft and launch costs also affect the cost of mission operations. Support teams
monitor spacecraft around the clock, to ensure continuing operation. At the first signs of failure,
a network of experts is assembled to ensure that the best expertise is available to deal with
ensuing problems. During normal operation, commands and instructions are carefully vetted to ensure
that they do not jeopardise the spacecraft’s performance, before they are communicated to the
spacecraft. Every aspect of the spacecraft operation is documented in minute detail, producing
hundreds of volumes of technical manuals that describe courses of action for all considered
eventualities. Communication with spacecraft is maintained during most phases of operation often as
a matter of course. For non-earth orbiting spacecraft this is particularly expensive, requiring the
use of large antennae in strategic locations around the world.
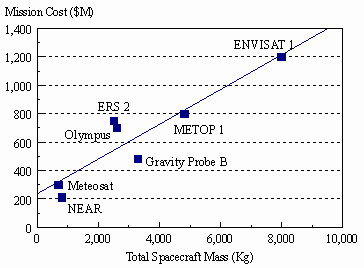
All of these contributing factors drive the costs of space programs into many millions of US
dollars. Mission costs can be broadly estimated from the mass of the spacecraft (Figure 1-1), small
spacecraft (around 1000 kg) costing hundreds of millions of dollars and large spacecraft (around
10000 kg) costing billions (Data from Jane's Space Directory). With such large programs, management
is extremely complex, and there is a tendency for costs to spiral. Mission failures and cost
over-runs are embarrassing for the organisations involved.
Recently, there has been considerable political pressure to reduce or to cap program costs,
shifting emphasis from scientific ideals to science value-for-money. A budding micro-satellite
industry offers budget priced access to space, providing a radical alternative to conventional
spacecraft development programmes, but is only suitable for missions with modest objectives. NASA
administrator Daniel Goldin announced his vision of "Faster, Better, Cheaper" conventional
missions, which led to NASA’s Discovery Program intended to "accomplish high quality, focused
planetary science, with innovative, streamlined, and efficient management approaches". The
approach limits design and manufacture to 18 months, with operations limited to three years. Mission
costs are capped to $185 million (fiscal year 1992).
Such new approaches to spacecraft design require new technologies to drive costs down while still
producing more reliable spacecraft. New techniques are needed to guard against system failure.
Increased spacecraft autonomy is required to allow the scaling down of ground activity and hence the
reduction of mission support costs. Spacecraft must make better use of fewer, lower quality
instruments and sensors to make them lighter and cheaper. NASA’s lead has sparked a general trend
towards smaller, more advanced spacecraft for science and technology missions that require less
expensive launch vehicles.
Next: Spacecraft Systems
References
 | Moss J. B., "Launch Vehicles", Spacecraft Systems Engineering, 2nd Edition,
ed. P. Fortescue and J. Stark, pp 145, John Wiley, 1995, ISBN 0-471-952206.
 | The Space review, Airclaims, June 1992.
 | "Long March Crash to End Insurance Discounts", Interspace, European Satellite
and Space News Ltd, pp 1 & 4, 21 February 1996.
 | Wilson A., "Launchers", Jane’s Space Directory, 11th Edition, Jane’s
Information Group Ltd, 1995, ISBN 0-7106-12591.
 | Fortescue P. and Stark J., Failures, Spacecraft Systems Engineering, 1st Edition, pp 396-397,
John Wiley, 1991, ISBN 0-471-927945.
 | Fortescue P. and Stark J., Spacecraft Systems Engineering, 1st Edition, pp 6, John
Wiley, 1991, ISBN 0-471-927945.
 | The Discovery Project Statement of Objectives, NASA policy statement, NASA,
http://www.nasa.gov,
1992. |
| | | | | |
|
|
|