1. Specialized CRISPR technologies for genome diversification
Genome engineering has revolutionized how we conduct biomedical research. This is largely due to the advances in the CRISPR-Cas system. At its core, CRISPR allows us to specifically and easily target any arbitrary sequence in the genome, a groundbreaking feat considering the sheer number of DNA base pairs in the human genome (3.2 billion).
The initial CRISPR technologies relied on creating double-stranded DNA breaks and error-prone DNA repair to modify sequences. This resulted in imprecise editing outcomes with limited utility. On the other hand, base editing is a newer genome editing technology that is more precise and does not involve creating breaks in the DNA. It uses nucleotide deaminases to convert single bases with high precision, acting as a molecular scalpel instead of a sledgehammer.
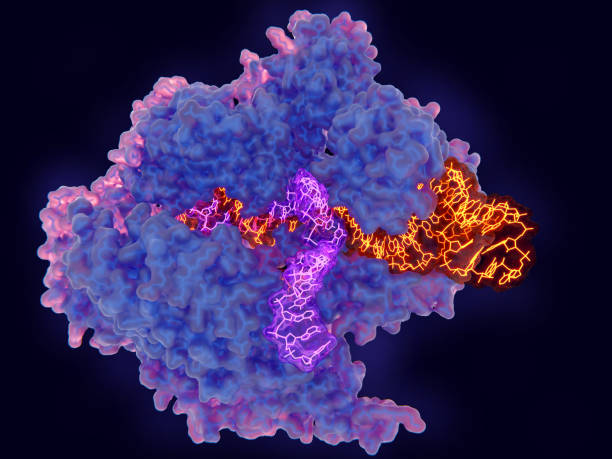
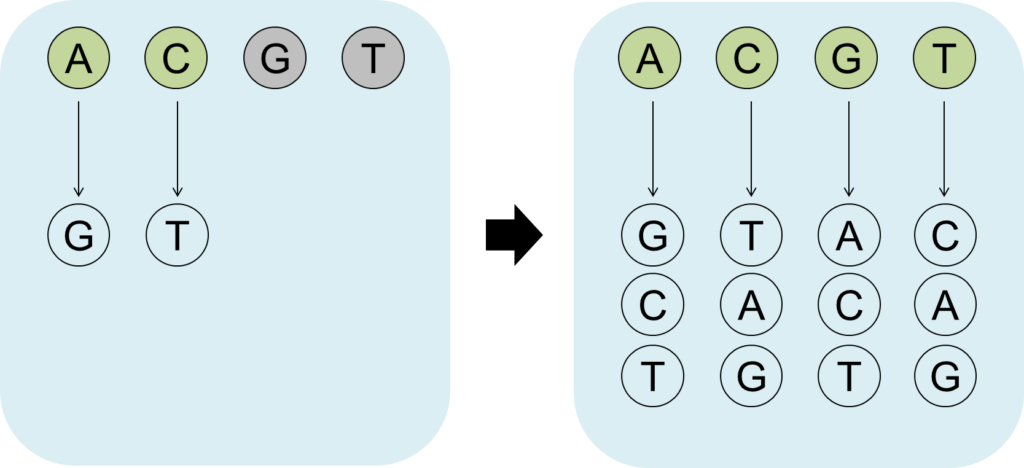
While there is a huge focus on improving genome editors for the precise installation of specific mutations, CRISPR can also be adopted towards creating targeted genetic diversity, where CRISPR creates a large number of different alleles in a defined location in the genome. These diversity-generating technologies allow us to identify de novo mutations and alleles with custom-tailored activities, even without the prior knowledge of the beneficial allele. For example, these technologies can be used during crop breeding to introduce new agricultural traits (e.g., larger fruits, accelerated growth, drought tolerance). In biomedical research, these technologies can facilitate functional genomics studies to identify and understand disease-causing mutations in key proteins.
Toward this goal, we are working on developing novel base editors with an expanded mutagenic scope. We use various protein engineering approaches to maximize their mutagenic potential especially in mammalian cells and higher-order organisms.
2. Engineering and understanding macromolecule stability in mammalian cells
Engineered macromolecules, including RNAs and proteins, are becoming an essential component of human therapeutics. They offer an unparalleled ability to modulate biological processes such as protein-protein interactions. However, RNAs and proteins must overcome a hostile cellular environment, where they are constantly recognized and degraded, making it challenging for functional macromolecules to accumulate at high enough concentration in the cell to create therapeutic benefit.
Recent advances in proteomics, genomics, and epigenomics have significantly improved our understanding of the factors that affect the intracellular stability of biomolecules. However, we have not yet effectively integrated these insights to create more potent functional biomolecules. To address this issue, we are employing high-throughput approaches to create custom stabilized RNAs and proteins and demonstrate their effectiveness in various biological settings. Advances in this research area will lead to general biomolecule design principles that will fuel the development of the next generation of macromolecular therapeutics.
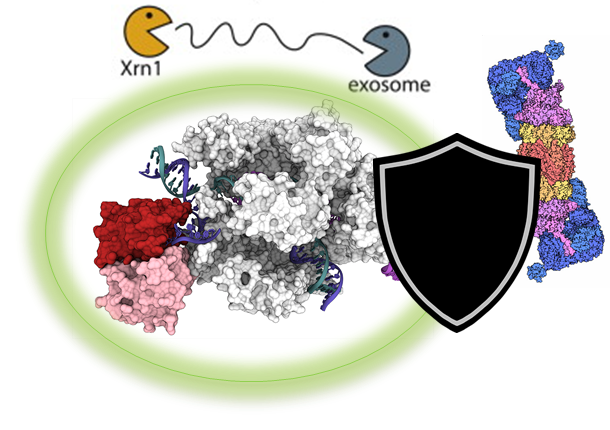